Introduction
The relationship between electricity and magnetism forms the basis of many modern technological devices. The discovery that an electric current can produce a magnetic field was first made by Hans Christian Oersted in 1820. This finding demonstrated that electric and magnetic fields are interrelated, laying the foundation for electromagnetism.
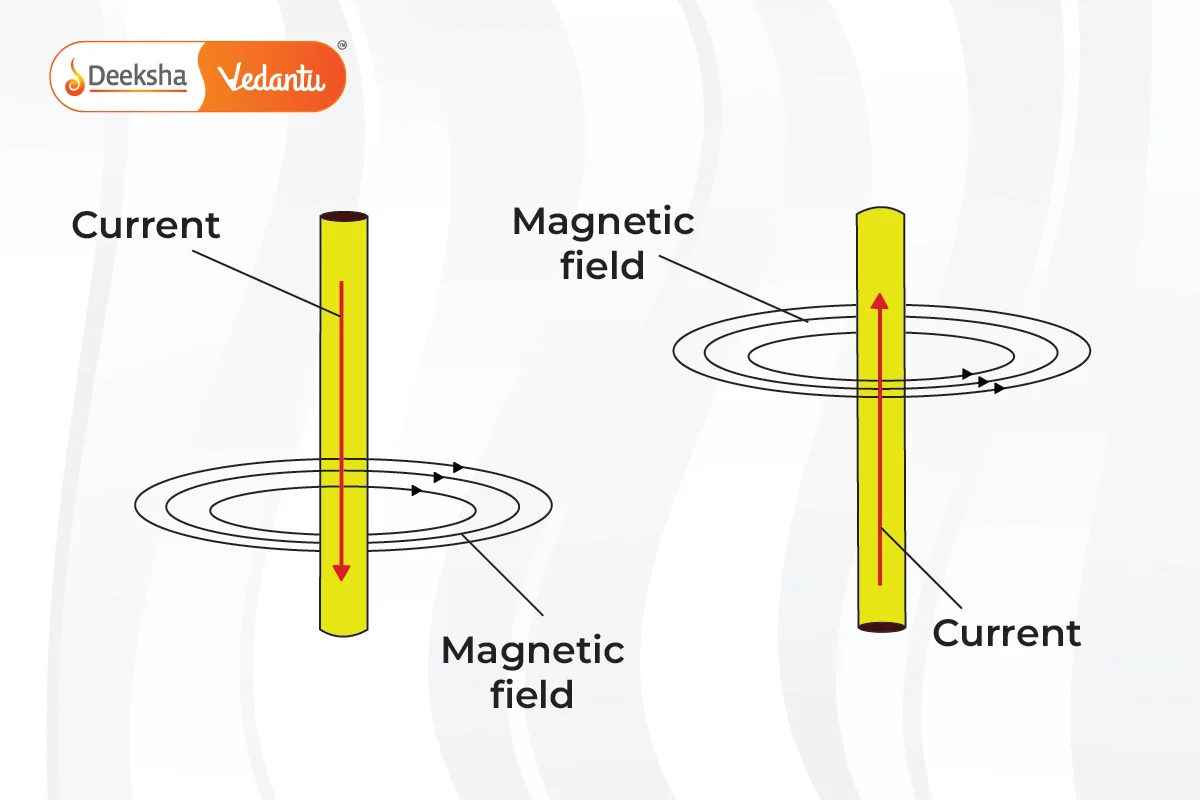
Key Concepts:
- When an electric current flows through a conductor (like a wire), it creates a magnetic field around it.
- This magnetic field can be detected using a compass or by observing the behavior of iron filings around the conductor.
- The direction and strength of the magnetic field depend on the current and the configuration of the conductor (straight, looped, or coiled).
Real-Life Application:
This principle is the foundation of devices such as electromagnets, electric motors, and generators.
Magnetic Field and Magnetic Field Lines
A magnetic field is the region around a magnet where a magnetic force can be detected. It is represented visually by magnetic field lines, which show the direction and strength of the magnetic field. Magnetic field lines provide a useful way to represent and understand the behavior of magnets and the magnetic effects of electric current.
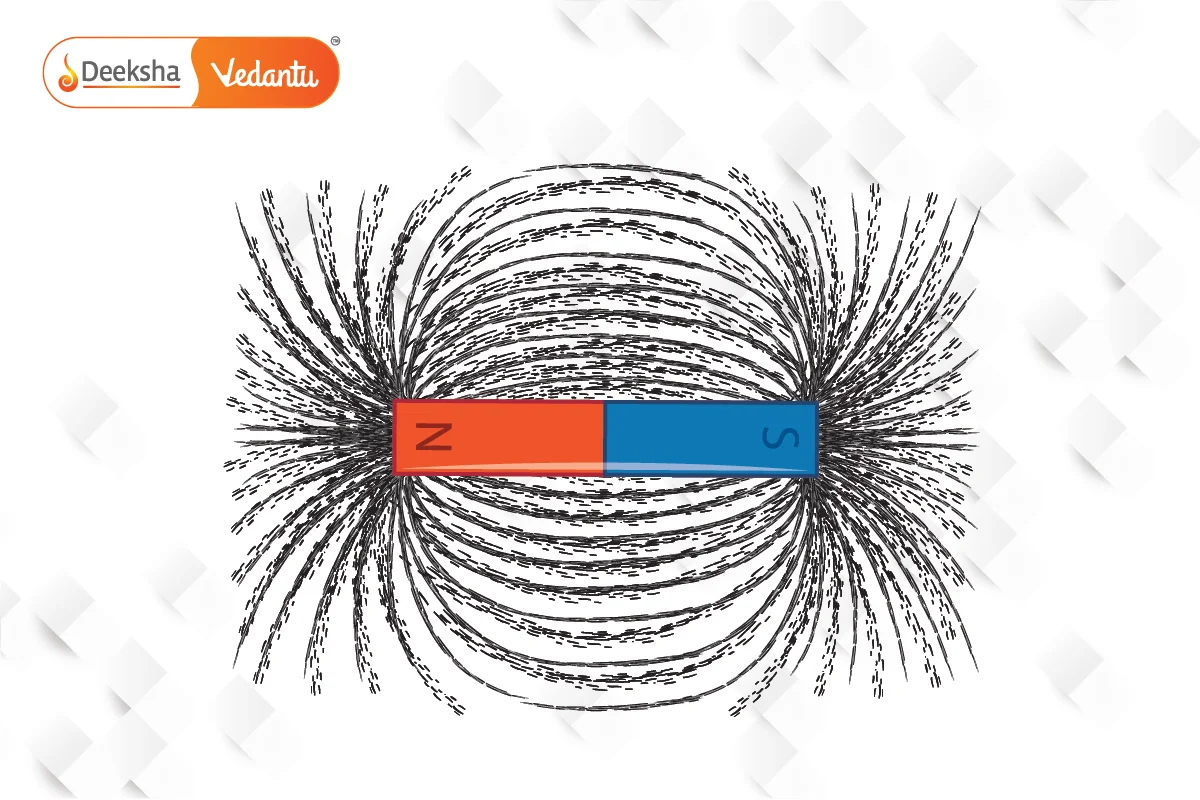
Properties of Magnetic Field Lines:
- Direction: Magnetic field lines emerge from the north pole and enter the south pole of a magnet.
- Closed Loops: Magnetic field lines form closed loops, meaning they continue through the magnet and complete a loop.
- Strength: The closer the field lines, the stronger the magnetic field at that point.
- Non-intersecting: Magnetic field lines never intersect because two magnetic field lines can’t have different directions at the same point.
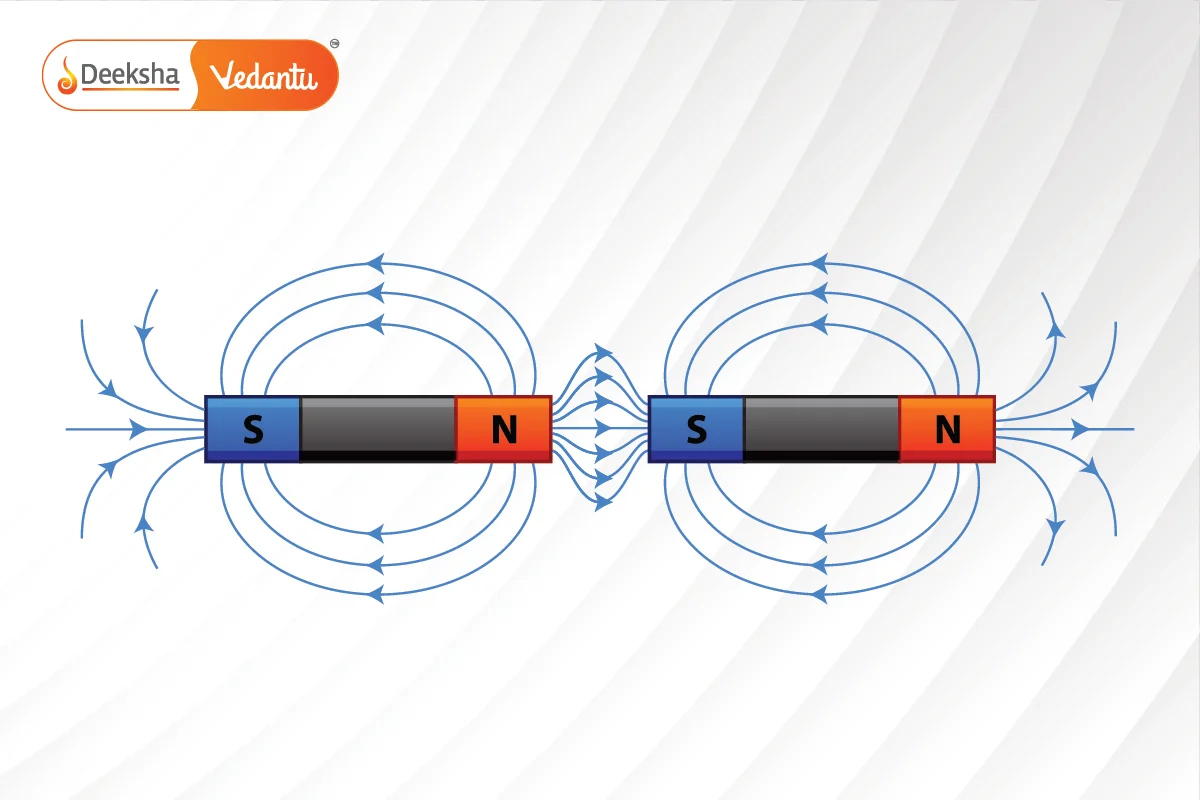
Example:
If you place a compass around a bar magnet, the needle aligns with the magnetic field lines. This alignment changes as you move the compass, revealing the invisible magnetic field lines.
Key Question:
- Q: Why do magnetic field lines never intersect?
- A: If two magnetic field lines intersected, it would imply that the magnetic field at the point of intersection has two directions, which is physically impossible.
Magnetic Field Due to a Current-Carrying Conductor
When an electric current flows through a conductor, such as a straight wire, it produces a magnetic field around it. The strength of this magnetic field depends on the amount of current flowing through the conductor and the distance from the wire.
Right-Hand Thumb Rule:
The Right-Hand Thumb Rule (or Ampere’s Right-Hand Rule) helps determine the direction of the magnetic field produced by a straight current-carrying conductor:
- Imagine holding the current-carrying conductor with your right hand, with the thumb pointing in the direction of the current. The direction in which your fingers curl represents the direction of the magnetic field around the conductor.
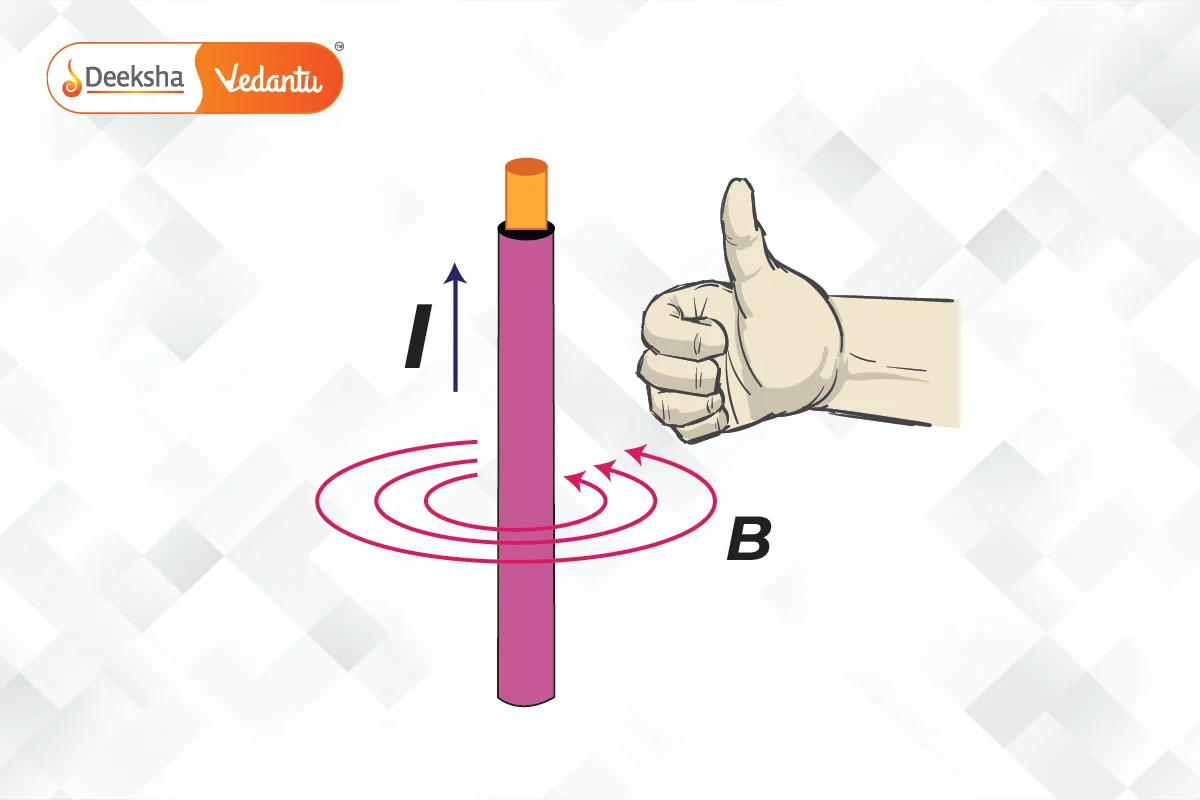
Magnetic Field Pattern:
- The magnetic field forms concentric circles around the wire, becoming weaker as you move further away from the wire.
- The direction of the magnetic field reverses when the direction of the current is reversed.
Factors Affecting the Magnetic Field Strength:
- Current: A stronger current results in a stronger magnetic field.
- Distance: The magnetic field strength decreases with increasing distance from the conductor.
Example:
- If a compass is placed near a current-carrying wire, the needle deflects, indicating the presence of a magnetic field.
Key Application:
- This principle is applied in magnetic compasses and electromagnets, where an electric current generates a controllable magnetic field.
Key Question:
- Q: What happens to the magnetic field if the current is increased?
- A: The strength of the magnetic field increases as the current increases.
Magnetic Field Due to a Circular Loop
When a current-carrying wire is bent into a circular loop, the magnetic field at any point on the loop behaves differently than it would in a straight wire.
Key Concepts:
- At each point on the loop, the magnetic field lines form concentric circles around the wire.
- Inside the loop, the magnetic field lines become nearly straight, reinforcing one another at the center of the loop.
- The strength of the magnetic field at the center of the loop increases with:
- The number of turns of the wire (more loops = stronger field).
- The current passes through the loop.
Magnetic Field at the Center of a Loop:
- The magnetic field produced by each segment of the loop adds up at the center, making the field stronger at the center than at any other point on the loop.
Example:
- Multiple loops (a coil) create an even stronger magnetic field. For instance, a solenoid is essentially a coil of wire, and it produces a strong, uniform magnetic field inside.
Key Question:
- Q: What happens to the magnetic field if the number of turns in a circular loop is doubled?
- A: Doubling the number of turns doubles the strength of the magnetic field at the center of the loop.
Magnetic Field Due to a Solenoid
A solenoid is a long coil of wire made up of several loops placed closely together. When an electric current passes through the solenoid, it produces a magnetic field similar to that of a bar magnet, with distinct north and south poles.
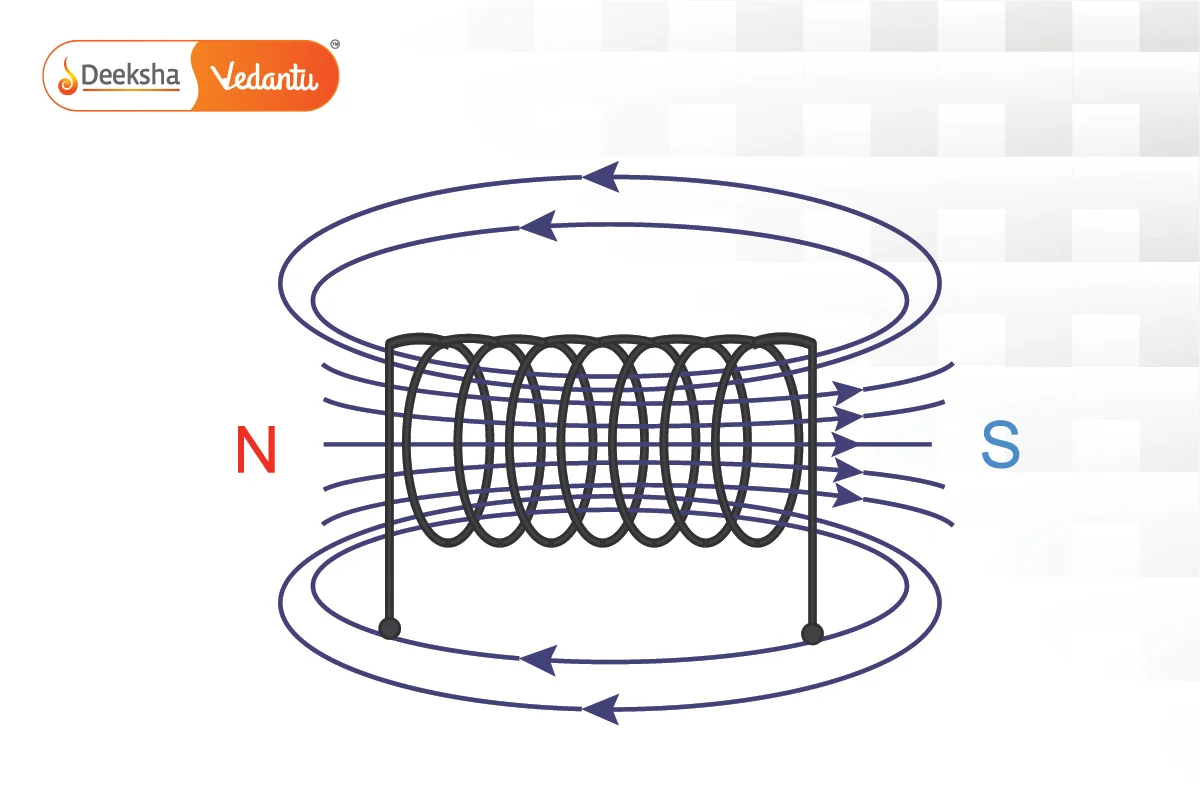
Magnetic Field Inside a Solenoid:
- The magnetic field inside a solenoid is strong, uniform, and directed along the axis of the solenoid.
- The strength of the magnetic field inside the solenoid can be increased by:
- Increasing the current through the solenoid.
- Increasing the number of turns of the wire in the solenoid.
- Inserting a soft iron core inside the solenoid, which increases the magnetic field strength dramatically.
Electromagnets:
When a soft iron core is placed inside the solenoid, it becomes an electromagnet—a magnet that can be switched on or off by controlling the current flowing through the solenoid.
Applications of Solenoids and Electromagnets:
- Electric bells: Electromagnets pull a metal striker to hit the bell when current flows.
- Magnetic cranes: Electromagnets lift heavy metal objects in scrap yards.
- MRI machines: Use electromagnets to generate strong magnetic fields for imaging.
Key Question:
- Q: How does increasing the current in a solenoid affect the magnetic field inside it?
- A: Increasing the current increases the strength of the magnetic field inside the solenoid.
Force on a Current-Carrying Conductor in a Magnetic Field
A current-carrying conductor placed in a magnetic field experiences a force. The direction and magnitude of this force depend on the direction of the current, the strength of the magnetic field, and the length of the conductor in the magnetic field.
Fleming’s Left-Hand Rule:
The direction of the force on the conductor can be determined using Fleming’s Left-Hand Rule:
- Stretch the thumb, forefinger, and middle finger of your left hand such that they are mutually perpendicular.
- The forefinger points in the direction of the magnetic field.
- The middle finger points in the direction of the current.
- The thumb indicates the direction of the force (motion) on the conductor.
Real-Life Application:
This principle is used in electric motors, where a current-carrying coil rotates when placed in a magnetic field, providing mechanical energy.
Key Example:
- A small aluminum rod placed between the poles of a magnet will move if a current flows through it, demonstrating the force exerted on the conductor.
Key Question:
- Q: What happens to the force on the conductor if the magnetic field strength is doubled?
- A: Doubling the magnetic field strength doubles the force on the conductor.
Electromagnetic Induction
Electromagnetic induction is the process by which a changing magnetic field induces an electric current in a conductor. This phenomenon was discovered by Michael Faraday in the early 19th century.
Faraday’s Law of Electromagnetic Induction:
Faraday’s Law states that the magnitude of the electromotive force (EMF) induced in a conductor is directly proportional to the rate of change of magnetic flux through the conductor. Mathematically, it is expressed as:
where:
is the magnetic flux through the conductor, defined as
,
is the magnetic field strength,
is the area of the conductor loop, and
is the angle between the magnetic field and the normal to the surface of the loop.
The negative sign indicates Lenz’s Law, which states that the induced EMF will oppose the change in magnetic flux that produced it.
Factors Affecting the Induced Current:
- Speed of movement: Faster motion induces more current.
- Strength of the magnetic field: A stronger magnetic field induces more current.
- Number of turns in the coil: More turns increase the induced current.
Applications of Electromagnetic Induction:
- Electric generators: Convert mechanical energy into electrical energy by rotating a coil in a magnetic field.
- Transformers: Change the voltage of alternating current (AC) using electromagnetic induction.
Key Example:
- A coil of wire moved through a magnetic field generates an induced current. If the motion stops, the current disappears.
Domestic Electric Circuits
In a domestic electrical circuit, appliances are connected to the main power supply. A live wire (positive, red) and a neutral wire (negative, black) form the basic connections, with an earth wire (green) providing safety.
Electric Fuse:
An electric fuse is a safety device that breaks the circuit if excessive current flows through it, preventing damage to appliances. The fuse wire melts when the current exceeds a certain limit, thereby protecting the circuit.
Circuit Breakers:
Modern homes often use circuit breakers, which automatically disconnect the circuit when a fault is detected, preventing electric shocks and fire hazards.
Key Application:
- Domestic appliances are wired in parallel, so they all receive the same voltage and can be operated independently.
Real-Life Applications of the Magnetic Effects of Electric Current
- Electric Motors: Convert electrical energy into mechanical energy, using the force on a current-carrying conductor in a magnetic field to rotate the motor.
- Electric Generators: Use electromagnetic induction to generate electricity by moving a conductor through a magnetic field.
- Transformers: Change the voltage of AC using the principles of electromagnetic induction.
- Magnetic Levitation Trains (Maglev): Use magnetic fields generated by electric currents to levitate and propel trains at high speeds.
Top Questions and Practice Problems
Q1: What is the direction of the magnetic field produced by a current-carrying conductor if the current flows from north to south?
- Answer: Using the right-hand thumb rule, the magnetic field forms concentric circles in the clockwise direction when viewed from above the conductor.
Q2: A solenoid with 150 turns is connected to a 12 V battery. What happens to the magnetic field if the number of turns is increased to 300, keeping the current constant?
- Answer: The magnetic field strength will double when the number of turns is doubled.
FAQs
An electric fuse protects appliances from damage by breaking the circuit if excessive current flows, preventing overheating and potential fires.
Fleming’s Left-Hand Rule is used to find the direction of force on a current-carrying conductor in a magnetic field. It’s applied in electric motors to understand the direction of motion.
Electromagnetic induction is used in devices like electric generators, transformers, and in technologies like magnetic levitation for high-speed trains (Maglev).
Electromagnetic induction is the process by which a changing magnetic field induces a current in a conductor. Michael Faraday discovered this phenomenon.
The magnetic field strength inside a solenoid increases as the number of turns increases, as each turn reinforces the magnetic field at the center of the solenoid.
Increasing the current increases the strength of the magnetic field around and at the center of the loop.
The magnetic field strength increases with a higher current and decreases as you move farther from the conductor.
Magnetic field lines never intersect because if they did, it would mean the magnetic field has two directions at the same point, which is physically impossible.
The Right-Hand Thumb Rule is used to determine the direction of the magnetic field around a straight current-carrying conductor. Point your right-hand thumb in the direction of the current, and your fingers will curl in the direction of the magnetic field lines.
A magnetic field around a conductor can be detected using a compass or by observing how iron filings arrange themselves around the conductor.
Hans Christian Oersted discovered in 1820 that an electric current can produce a magnetic field, revealing the relationship between electricity and magnetism.
When an electric current flows through a conductor, it creates a magnetic field around it. This magnetic effect is the basis for devices like electromagnets, electric motors, and generators.
Related Topics
- Physics FAQs
- Pressure
- Difference between AC and DC
- Electric Power
- Bernoullis Principle
- Force
- Thermodynamics
- Kirchhoff’s Law
- Laws of Motion
- What is Hypothesis?
- Wheatstone Bridge
- Atmospheric Refraction
- Ohm’s Law
- Magnetic Field Due To A Current – Carrying Conductor
- Factors On Which The Resistance Of A Conductor Depends
Get Social