Introduction
A magnetic field is a region around a magnetic material or a moving electric charge where the force of magnetism acts. It is an invisible area in which magnetic forces can be detected and influence other magnetic materials or charged particles. Magnetic fields are created either by magnets or by electric currents.
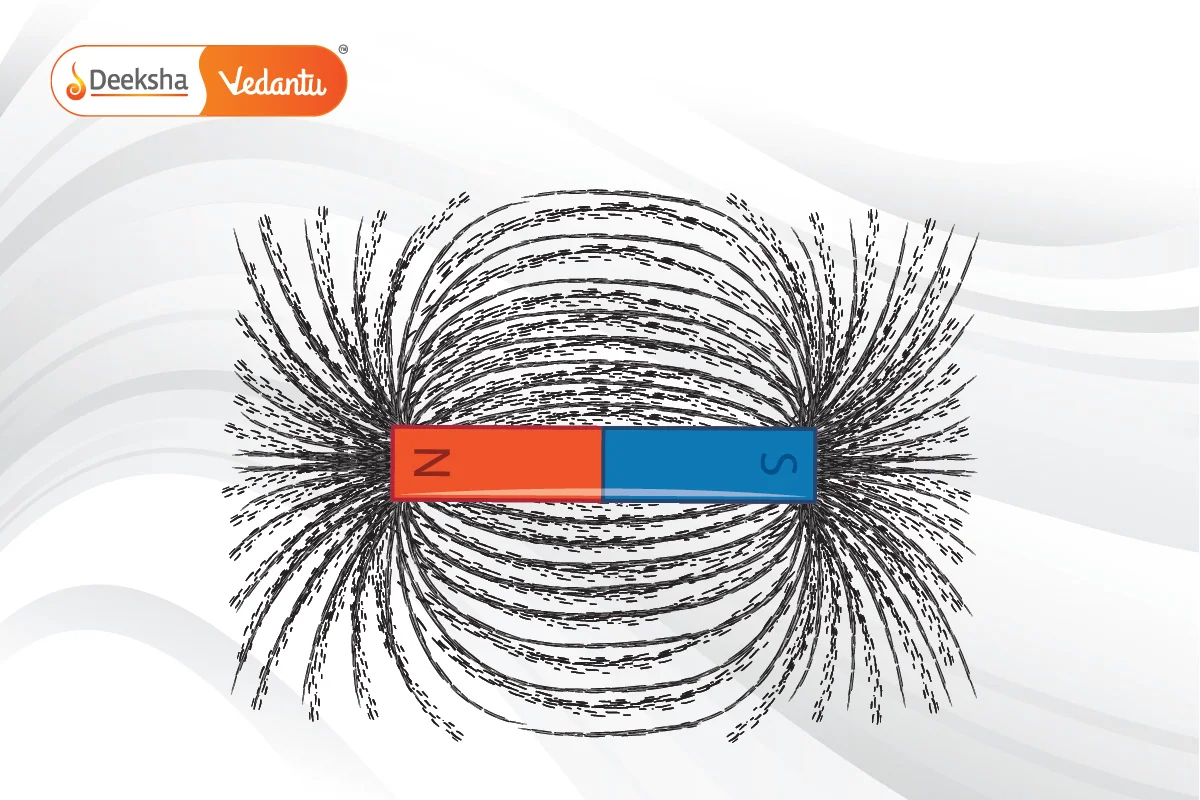
In daily life, we observe magnetic fields in several ways. For example, a compass needle points toward the north due to the Earth’s magnetic field. Electric currents in power lines generate magnetic fields that surround the wires.
Understanding magnetic fields is crucial in many technological applications, including electric motors, generators, and transformers, which utilize the magnetic effects of electric current.
What is a Magnetic Field?
A magnetic field is defined as the region around a magnet or a current-carrying conductor in which magnetic forces can be observed. The strength of the magnetic field depends on the distance from the magnet or current and the nature of the material.
Visualizing Magnetic Fields:
Magnetic fields are often represented using magnetic field lines, which are imaginary lines used to depict the direction and strength of the field. The behavior of these lines around a magnet helps us understand the forces at play in the magnetic field.
The magnetic field produced by a bar magnet can be visualized by placing a compass or iron filings near the magnet. The pattern formed by the filings provides a visual representation of the magnetic field lines.
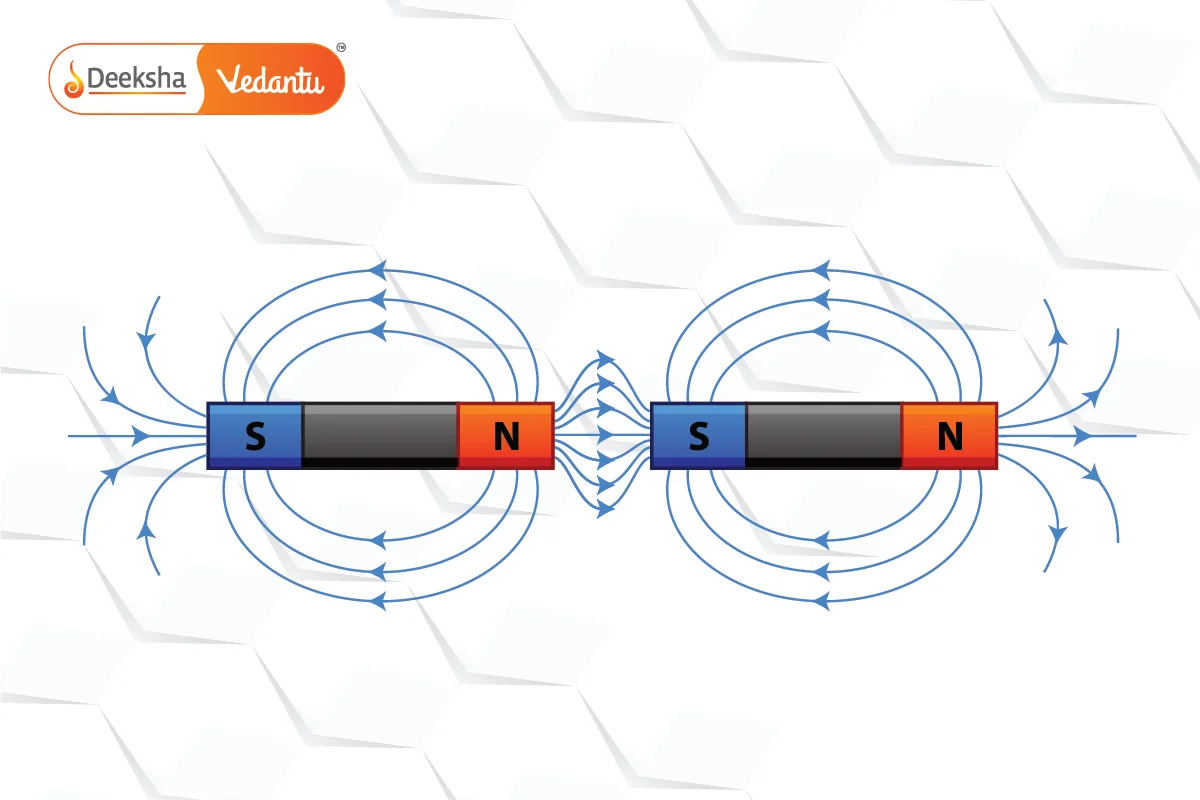
Magnetic Field Lines: Definition and Key Properties
Magnetic field lines are imaginary lines used to represent the strength and direction of a magnetic field. These lines allow us to map out how the magnetic force behaves around a magnet or a current-carrying conductor.
Key Properties of Magnetic Field Lines:
- Closed Loops: Magnetic field lines always form closed loops. Outside a magnet, they travel from the north pole to the south pole. Inside the magnet, they move from the south pole to the north pole, completing the loop.
- Non-Intersecting: Magnetic field lines never intersect. If they did, it would imply that the magnetic field has two directions at the same point, which is physically impossible.
- Direction: The direction of the magnetic field at any point is tangent to the field line at that point. This direction is indicated by arrows on the field lines, pointing from the north pole to the south pole outside the magnet.
- Strength of the Field: The closer the magnetic field lines are to each other, the stronger the magnetic field at that location. Conversely, the farther apart the lines are, the weaker the field.
- Field Lines Around a Bar Magnet: Around a bar magnet, magnetic field lines emerge from the north pole, curve around the magnet, and enter the south pole. Inside the magnet, they continue from the south pole to the north pole.
Magnetic Field Lines Around Different Magnets
Magnetic Field of a Bar Magnet:
- A bar magnet produces a magnetic field where the lines emerge from the north pole and curve around to enter the south pole. Inside the magnet, the field lines move from the south pole to the north pole, forming a continuous loop.
- Example: If you place a compass near the north pole of a bar magnet, the compass needle aligns with the magnetic field lines, showing the direction of the field at that point.
Magnetic Field Around a Circular Loop:
- When current flows through a circular loop, magnetic field lines form concentric circles around the wire. Inside the loop, the magnetic field is stronger and more uniform.
Key Point: The strength of the magnetic field increases as the number of turns in the loop increases. The more loops, the stronger the magnetic field inside the loop.
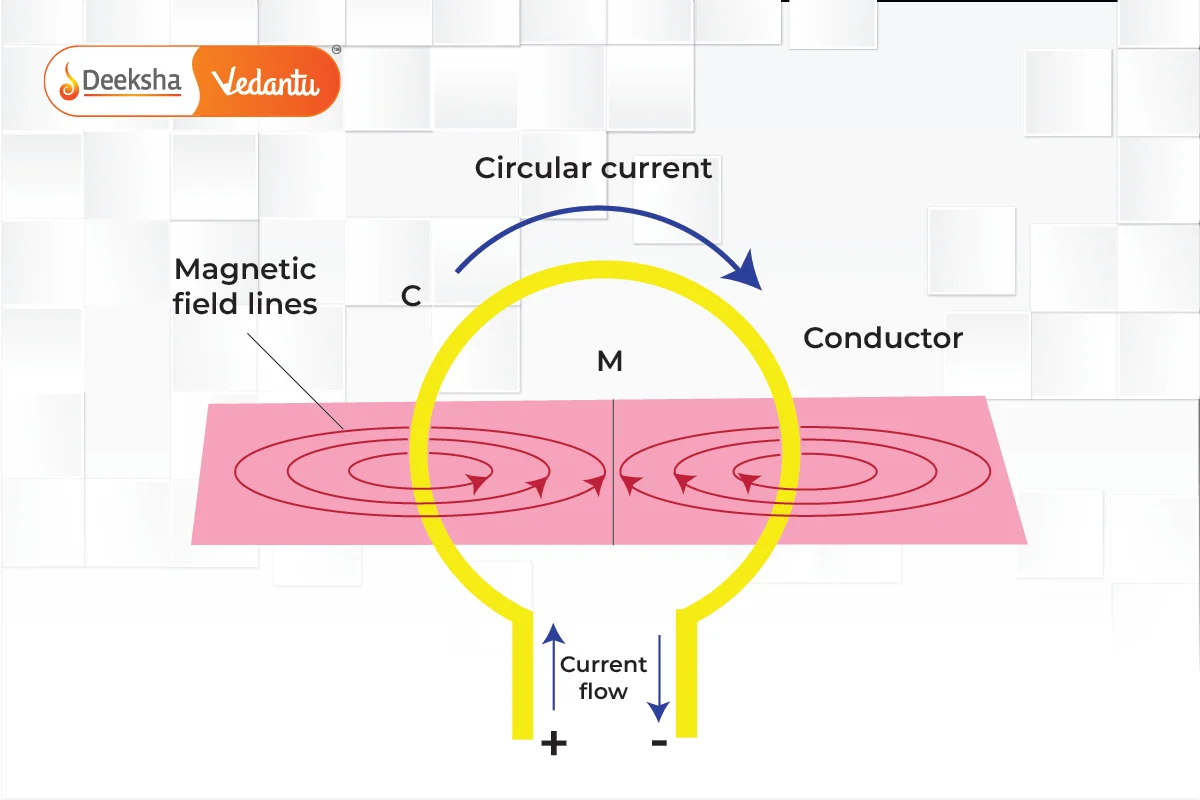
Magnetic Field of a Solenoid:
- A solenoid is a long coil of wire, where current flows through multiple loops. The magnetic field inside a solenoid is strong, uniform, and similar to that of a bar magnet. The field lines inside the solenoid are parallel and straight, indicating a strong and uniform field.
Key Application: Solenoids are used in electromagnets. By inserting a soft iron core inside the solenoid, you can create a powerful electromagnet used in devices like electric bells, motors, and magnetic cranes.
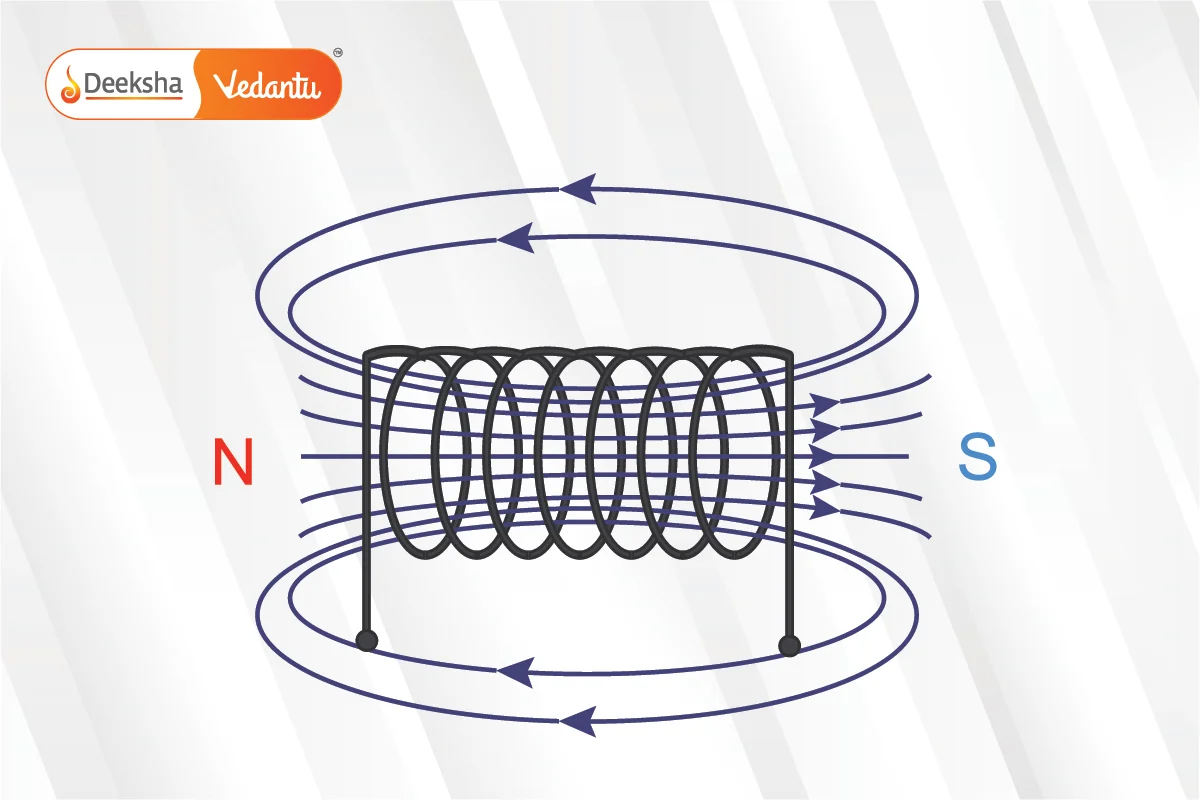
Oersted’s Experiment
The connection between electricity and magnetism was discovered by the Danish physicist Hans Christian Oersted in 1820. He conducted a simple experiment that revealed that a current-carrying conductor produces a magnetic field.
Oersted’s Experiment Setup:
- Oersted placed a compass needle near a straight wire through which electric current was passed.
- He observed that the compass needle deflected when the current flowed through the wire, and the direction of deflection changed when the direction of the current was reversed.
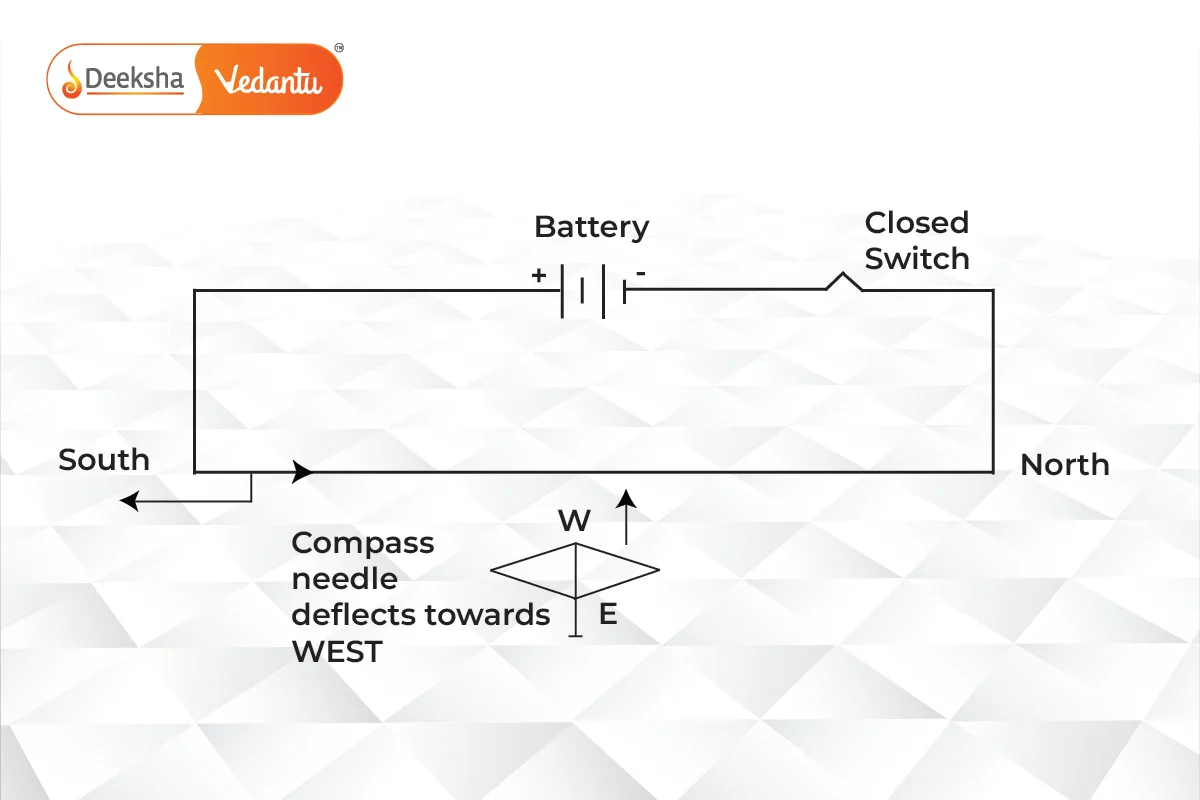
Conclusion of the Experiment:
Oersted concluded that a current-carrying conductor produces a magnetic field around it. This discovery laid the foundation for electromagnetism, which is the basis for the functioning of electric motors, generators, transformers, and various other devices.
The Right-Hand Thumb Rule
To determine the direction of the magnetic field produced by a current-carrying straight conductor, we use the Right-Hand Thumb Rule.
- Imagine holding a current-carrying conductor in your right hand, with your thumb pointing in the direction of the current. The direction in which your fingers curl around the conductor gives the direction of the magnetic field lines.
Application: This rule helps us understand how the magnetic field behaves around a current-carrying wire. For example, if the current flows vertically upward through a wire, the magnetic field will form concentric circles around the wire, with the field direction determined by the right-hand thumb rule.
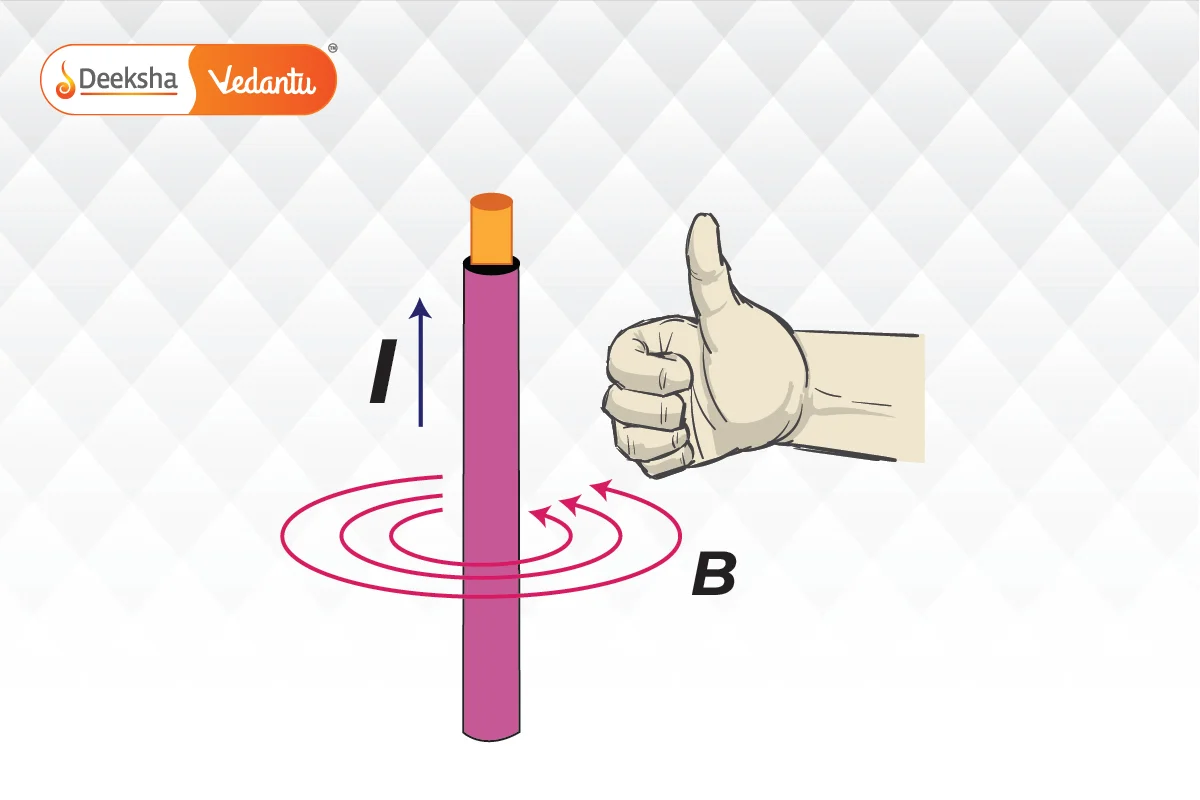
Example:
- If a current flows from north to south in a straight wire, the right-hand thumb rule indicates that the magnetic field lines will form concentric circles in a clockwise direction around the wire when viewed from above.
Earth’s Magnetic Field
The Earth behaves like a giant bar magnet, with its own magnetic field. The magnetic north pole and magnetic south pole are not exactly aligned with the geographical poles, but they are close. The Earth’s magnetic field is what causes a compass needle to align itself with the north and south directions.
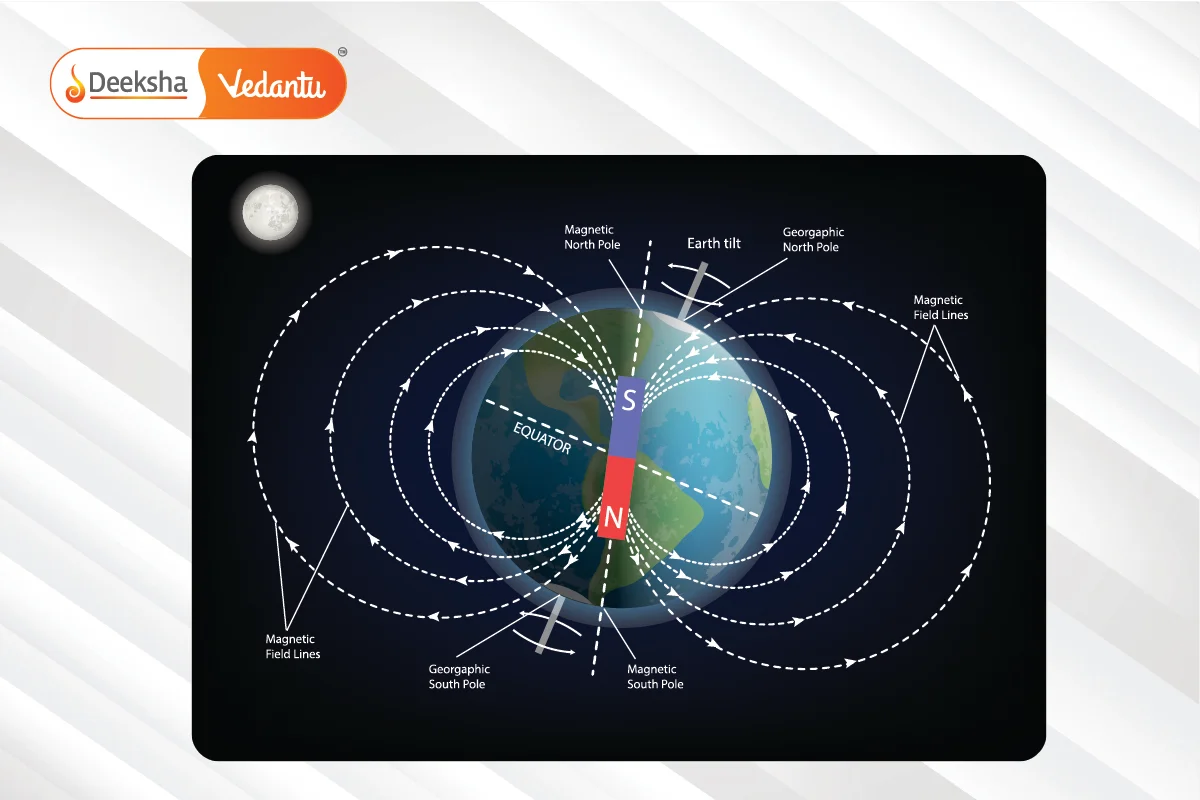
Properties of Earth’s Magnetic Field:
- The Earth’s magnetic field lines run from the south magnetic pole to the north magnetic pole outside the Earth, just like a bar magnet. Inside the Earth, the lines run from the north magnetic pole to the south magnetic pole.
Practical Application:
- Magnetic compasses: A compass needle aligns with the Earth’s magnetic field, pointing towards the magnetic north. This principle is used in navigation to find directions.
Real-Life Applications of Magnetic Fields
Magnetic fields and their properties are applied in various real-world technologies, making them indispensable in modern life:
Electric Motors: Electric motors use magnetic fields to convert electrical energy into mechanical energy. A current-carrying coil is placed in a magnetic field, and the interaction between the magnetic field and the current causes the coil to rotate, generating mechanical work.
Magnetic Resonance Imaging (MRI): MRI machines use strong magnetic fields to create detailed images of the body’s internal organs and tissues. This non-invasive imaging technique is crucial in medical diagnostics.
Magnetic Cranes: Electromagnets are used in large cranes to lift and move heavy metal objects, such as scrap metal. The magnetic field is generated when current flows through the electromagnet, allowing it to attract metal objects.
Transformers: Transformers work on the principle of electromagnetic induction and are used to step up or step down the voltage in AC circuits. They play a crucial role in power transmission.
Key Practice Questions and Problems
Q1: Draw the magnetic field lines around a bar magnet. What is the direction of these lines outside and inside the magnet?
- Answer: The magnetic field lines outside a bar magnet emerge from the north pole and curve around to enter the south pole. Inside the magnet, they move from the south pole to the north pole, forming closed loops.
Q2: How can you demonstrate the presence of a magnetic field around a current-carrying conductor?
- Answer: You can demonstrate the magnetic field around a current-carrying conductor by placing a compass near the conductor. When current flows through the conductor, the compass needle will deflect, indicating the presence of a magnetic field.
Q3: Explain what happens to the strength of the magnetic field as you move farther away from a bar magnet.
- Answer: The strength of the magnetic field decreases as you move farther away from the bar magnet. The magnetic field lines become more spread out, indicating that the field is weaker at greater distances.
Q4: What will happen to the magnetic field if the current through a straight conductor is doubled?
- Answer: If the current through a straight conductor is doubled, the strength of the magnetic field around the conductor will also increase. The magnetic field is directly proportional to the current.
FAQs
Around a bar magnet, magnetic field lines emerge from the north pole, curve around the magnet, and enter the south pole. Inside the magnet, the lines continue from the south pole to the north pole, forming closed loops.
You can visualize magnetic field lines by sprinkling iron filings around a magnet or by using a small compass. The iron filings align themselves along the magnetic field lines, forming a pattern that reveals the field’s shape and direction.
Magnetic field lines are imaginary lines used to represent the strength and direction of a magnetic field. They help us visualize how the magnetic force behaves around a magnet or a current-carrying conductor.
An electric fuse protects appliances from damage by breaking the circuit if excessive current flows, preventing overheating and potential fires.
Fleming’s Left-Hand Rule is used to find the direction of force on a current-carrying conductor in a magnetic field. It’s applied in electric motors to understand the direction of motion.
Electromagnetic induction is used in devices like electric generators, transformers, and in technologies like magnetic levitation for high-speed trains (Maglev).
Electromagnetic induction is the process by which a changing magnetic field induces a current in a conductor. Michael Faraday discovered this phenomenon.
The magnetic field strength inside a solenoid increases as the number of turns increases, as each turn reinforces the magnetic field at the center of the solenoid.
Increasing the current increases the strength of the magnetic field around and at the center of the loop.
The magnetic field strength increases with a higher current and decreases as you move farther from the conductor.
Magnetic field lines never intersect because if they did, it would mean the magnetic field has two directions at the same point, which is physically impossible.
The Right-Hand Thumb Rule is used to determine the direction of the magnetic field around a straight current-carrying conductor. Point your right-hand thumb in the direction of the current, and your fingers will curl in the direction of the magnetic field lines.
A magnetic field around a conductor can be detected using a compass or by observing how iron filings arrange themselves around the conductor.
Hans Christian Oersted discovered in 1820 that an electric current can produce a magnetic field, revealing the relationship between electricity and magnetism.
When an electric current flows through a conductor, it creates a magnetic field around it. This magnetic effect is the basis for devices like electromagnets, electric motors, and generators.
Get Social